By means of
NASA’s James Webb Space Telescope examines potentially habitable exoplanets in our Milky Way, focusing on small planets that could support life due to their location in the habitable zone. Detecting atmospheres, let alone biosignatures indicative of life, is extremely challenging due to the minuscule size of the signals from these planets and the interference from their host stars. Credit: SciTechDaily.com
The James Webb Space Telescope actively researches small, potentially habitable exoplanets, using transmission spectroscopy to analyze their atmospheric composition. The process is complicated by small signal sizes and the need for extended observation times, making the detection of life’s biosignatures a demanding task.
Exoplanets are common in our Milky Way, and some even orbit their star’s so-called habitable zone. NASA’s James Webb Space Telescope has been busy observing some of these small, potentially habitable planets, and astronomers are now hard at work analyzing Webb data. NASA invited Drs. Knicole Colón and Christopher Stark, two Webb project scientists at NASA’s Goddard Space Flight Center, to tell us more about the challenges of studying these other worlds.
Defining potentially habitable planets
“A potentially habitable planet is often defined as a planet similar in size to Earth that orbits within its star’s ‘habitable zone’, a location where the planet could be have a temperature at which liquid water is could be exist on the surface. We currently know of about 30 planets that may be small, rocky planets like Earth, orbiting in the habitable zone.
“However, there is no guarantee that a planet orbiting in the habitable zone is actually habitable (it could support life), let alone inhabited (it currently supports life). At the time of writing, there is only one known habitable and inhabited planet: Earth!
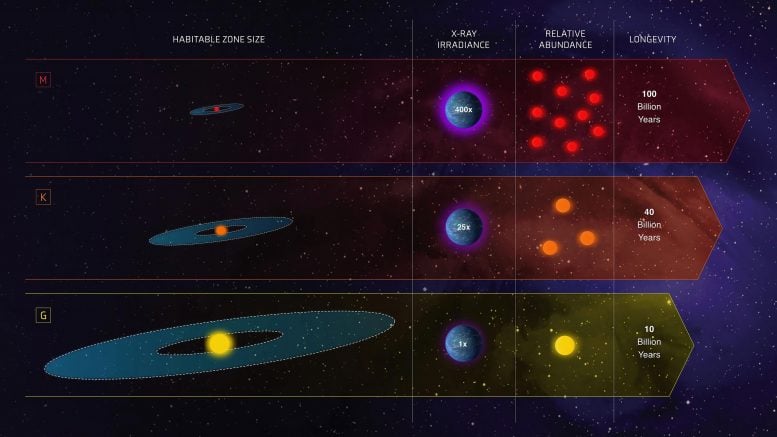
This infographic compares the characteristics of three classes of stars in our Milky Way: Sun-like stars are classified as G stars; stars that are less massive and cooler than our Sun are K dwarfs; and even fainter and cooler stars are the reddish M dwarfs. The size of the habitable zone is different for each class of stars. In our solar system, the habitable zone begins just beyond the orbit of Venus and nearly includes Mars. Credit: NASA, ESA and Z. Levy (STScI)
Challenges in observing exoplanet atmospheres
“The potentially habitable worlds Webb observes are all transiting exoplanets, meaning their orbits are almost sideways, so they pass in front of their guest stars. Webb takes advantage of this orientation to perform transmission spectroscopy as the planet passes in front of its star. This orientation allows us to examine the starlight filtered through the atmospheres of planets to learn more about their chemical composition.
“However, the amount of starlight blocked by the thin atmosphere of a small rocky planet is small, typically much less than 0.02%. Simply detecting an atmosphere around these small worlds is quite a challenge. Identifying the presence of water vapor, which can increase the possibility of habitability, is even more difficult. Seeking biosignatures (biologically produced gases) is extremely difficult, but also an exciting endeavor.
When a exoplanet passes directly between its host star and the observer, we say that the planet passes in front of its host star. This transit dims the star’s light to a measurable degree, and starlight is also filtered through the exoplanet’s atmosphere, if it has one. This animation shows a single planet and the corresponding change in light levels during its transit. Credit: NASA’s Jet Propulsion Laboratory
“There are currently only a handful of small, potentially habitable worlds considered accessible for atmospheric characterization with Webb, including the planets LHS 1140 b and TRAPPIST-1 e.
Technical challenges in detecting biosignatures
“Some recent theoretical work investigating the detectability of gaseous molecules in the atmosphere of the super-Terrestrial planet LHS 1140 b highlights several challenges in the search for biosignatures. The work notes that about 10 to 50 transits of the planet around its parent star would be needed, equivalent to 40 to 200 hours of observation time with Webb, to try to detect potential biosignatures such as ammonia, phosphine, chloromethane and nitrous oxideat best of a clear, cloud-free atmosphere.
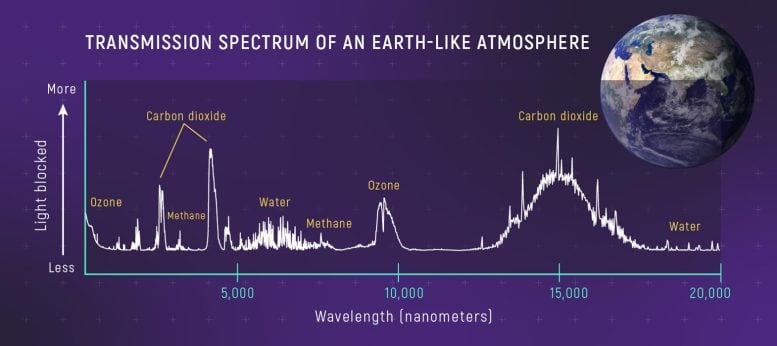
A simulated transmission spectrum of an Earth-like atmosphere shows wavelengths of sunlight absorbing molecules such as ozone (O3), water (H2O), carbon dioxide (CO2), and methane (CH4). (Note that in this graph, the y-axis represents the amount of light blocked by the Earth-like planet’s atmosphere rather than the brightness of sunlight traveling through the atmosphere: brightness decreases from bottom to top. ) Model transmission spectrum from Lisa Kaltenegger and Zifan Lin 2021 ApJL 909. Credit: NASA, ESA, Leah Hustak (STScI)
The complexity of exoplanet observing schemes
“Since Webb cannot view the LHS 1140 system year-round due to the system’s location in the sky, it would take several years, if not nearly a decade, to collect 50 transit observations of LHS 1140 b. The search for biosignatures may even require more than 50 transit observations if the planet’s atmosphere is cloudy.
“Most small exoplanets are known to have clouds or nebulae that dampen or obscure the signal they are looking for. The atmospheric signals from these biosignature gases also tend to overlap with other expected atmospheric signals (e.g. due to gaseous methane or carbon dioxide), so distinguishing between the different signals is another challenge.
Hycean Planets: a new avenue for research
“One possible avenue in the search for biosignatures is the study of Hycean planets, a theoretical class of super-Earth-sized planets with a relatively thin hydrogen-rich atmosphere and a significant liquid water ocean. The super-Earth K2-18 b is a candidate for a potentially habitable Hycean planet based on current data from Webb and other observatories.
“Recently published work used NIRSpec and NIRISS to detect methane and carbon dioxide in K2-18 b’s atmosphere, but not in water. This means that the suggestion that K2-18 b is a Hycean world with a liquid water ocean remains based on theoretical models, without direct observational evidence. The authors of the work also pointed out the possible presence of the potential biosignature dimethyl sulfide in the atmosphere of K2-18 b, but the potential dimethyl sulfide signal is too weak for a conclusive detection in the current data.
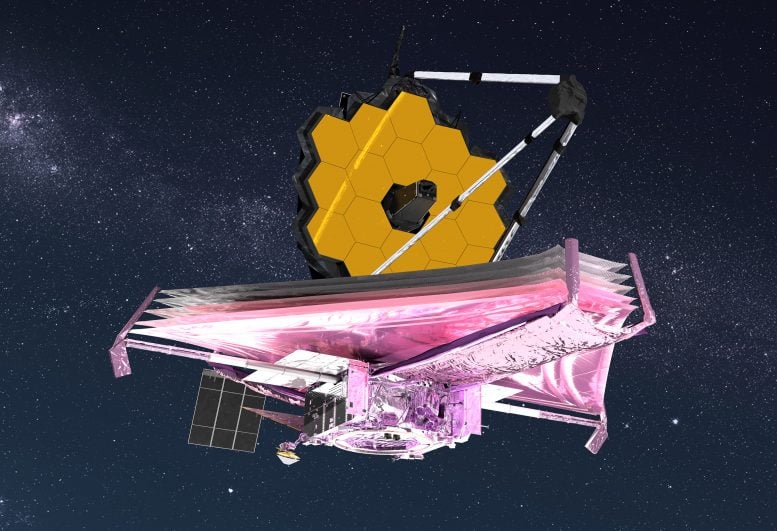
Artistic conception of the James Webb Space Telescope. Credit: NASA GSFC/CIL/Adriana Manrique Gutierrez
“The concept and study of the Hycean planet class is very new, so alternative interpretations of the liquid water ocean scenario (and thus of the potential for a habitable environment) are still being explored. Upcoming Webb observations with the NIRSpec and MIRI instruments should shed further light on the nature of the potential Hycean planet K2-18 b and the possible presence of dimethyl sulfide in its atmosphere.
Confounding factors in observational data
“Another confounding factor that makes Webb’s research into small, potentially habitable worlds challenging is that the host stars may also show signs of water vapor. This was explored in recent Webb observations of the rocky exoplanet known as GJ 486 b. We therefore have the additional challenge of determining whether the water vapor detected by Webb actually comes from a planet’s atmosphere and not from its star.
Conclusion: The future of exoplanet studies
“The detection of biosignatures in the atmospheres of small, potentially habitable planets orbiting cool stars is an extremely challenging endeavor, typically requiring ideal conditions (e.g., a cloud-free atmosphere) or assuming early Earth environments (i.e. , different from that of modern Earth). we know), the detection of signals significantly smaller than 200 parts per million, a well-behaved star with no significant water vapor on starspots, and a significant amount of telescope time to achieve sufficient signal-to-noise.
“It is also important to keep in mind that the detection of a single biosignature, by any means, does not constitute a discovery of life. The discovery of life on an exoplanet will likely require a large number of unambiguously detected biosignatures, data from multiple missions and observatories, and extensive atmospheric modeling efforts, a process that will likely take years.
“Webb’s strength is that it has the sensitivity to detect and characterize the atmospheres of a handful of the most promising potentially habitable planets orbiting cool stars. In particular, Webb has the ability to detect a range of molecules important to life, such as water vapor, methane and carbon dioxide. Our goal is to learn as much as possible about worlds that could potentially be habitable, even if we cannot definitively identify habitable features with Webb.
“Webb observations, combined with exoplanet studies by NASA’s upcoming Nancy Grace Roman Space Telescope, will ultimately lay the foundation for the future Habitable Worlds Observatory, which will be NASA’s first mission purpose-built to directly image chemical trails caused by life on Earth bring and search. Earth-like planets around sun-like stars.”
About the Authors:
Knicole Colón is an astrophysicist at NASA’s Goddard Space Flight Center in Greenbelt, Maryland, and serves as the Deputy Project Scientist for the James Webb Space Telescope for Exoplanet Science.
Christopher Stark is an astrophysicist in the Exoplanets and Stellar Astrophysics Laboratory at NASA’s Goddard Space Flight Center in Greenbelt, Maryland, and serves as deputy observatory project scientist for the James Webb Space Telescope.