The simple hagfish is an ugly, gray, eel-like creature best known for its ability to release a cloud of sticky slime on unsuspecting predators, clogging the gills and suffocating the predators. That is why it is also affectionately called a ‘snot snake’. Hagfish also like to burrow into deep-sea sediment, but scientists have not been able to observe exactly how they do this because the cloudy sediment obscures visibility. Researchers at Chapman University built a special tank of transparent gelatin to overcome this challenge and get a complete picture of burrowing behavior, according to a new paper published in the Journal of Experimental Biology.
“We’ve known for a long time that hagfish can burrow into soft sediments, but we had no idea how they do it,” said co-author Douglas Fudge, a marine biologist who directs a laboratory at Chapman studying hagfish. “By figuring out how to get hagfish to voluntarily burrow into transparent gelatin, we were able to gain insight into this process for the first time.”
As previously reported, scientists have been studying mucus from hagfish for years because it is such an unusual material. It’s not like slime, which dries out and hardens over time. Hagfish slime remains slimy, giving it the consistency of semi-solidified gelatin. This is due to the long, thread-like fibers in the mucus, in addition to the proteins and sugars that make up mucin, the other important component. These fibers roll up into ‘strands’ that resemble balls of yarn. When the hagfish releases a shot of slime, the strands uncoil and combine with the salt water, causing them to inflate more than 10,000 times their original size.
From a material point of view, slime slime is a fascinating stuff that could one day be useful for biomedical devices, or for weaving light but strong fabrics for natural lycra or body armor, or for lubricating industrial drills that tend to to become hidden in deep soil and sediment. In 2016, a group of Swiss researchers studied the unusual liquid properties of hagfish mucus, focusing specifically on how these properties provided two distinct benefits: helping the animal defend itself against predators and tying itself into knots to use its own mucus to escape.
Hagfish slime is a non-Newtonian fluid and is unusual in that it has both a shear-thickening and a shear-thinning action. Most hagfish predators use suction feeding, which creates a unidirectional shear thickening flow, which improves the gills’ ability to clog and suffocate the predators. But when the hagfish needs to get out of its own slime, its body movements create a current that thins, causing the slimy network of cells that make up the slime to collapse.
Fudge has been studying the hagfish and the properties of its slime for years. For example, in 2012, while at the University of Guelph, Fudge’s lab successfully harvested hagfish mucus, dissolved it in liquid, and then “spun” it into a strong, yet stretchy thread, much like spinning silk. It is possible that such threads could replace the petroleum-based fibers currently used in hard hats or Kevlar vests, among other possible applications. And in 2021, his team discovered that the slime produced by larger hagfishes contains much larger cells than the slime produced by smaller hagfishes – an unusual example of the scaling of cell size with body size in nature.
A sedimentary solution
This time, Fudge’s team has turned their attention to digging hagfish. In addition to shedding light on the reproductive behavior of hagfish, the research could also have broader ecological implications. According to the authors, burrowing is an important factor in sediment turnover, while burrow ventilation changes the chemistry of the sediment so that it can hold more oxygen. This would in turn change which organisms are likely to thrive in that sediment. Understanding the digging mechanisms could also help in the design of soft digging robots.
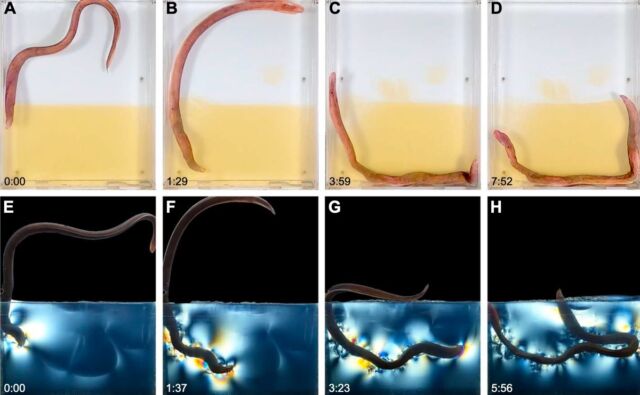
DS Fudge et al., 2024
But first Fudge’s team had to figure out how to see through the sediment to observe the burrowing behavior. Other scientists studying different animals have relied on transparent substrates such as mineral cryolite or hydrogels made from gelatin, the latter of which has been successfully used to observe the burrowing behavior of bristleworms. Fudge et al. chose gelatin as a sediment replacement, housed in three custom-made transparent acrylic chambers. They then filmed the gelatin-burrowing behavior of 25 randomly selected hagfish.
This allowed Fudge et al. to identify two different phases of movement that the hagfish used to create their u-shaped burrows. First, there is the “thrash” phase, during which the hagfish swims vigorously while moving its head back and forth. This not only serves to propel the hagfish forward, but also helps to break up the gelatin. This may be how hagfish overcome the challenge of creating an opening in the sediment (or gelatin substrate) for them to move through.
Next comes the “coil phase”, which appears to be driven by an “internal concertina” common in snakes. It involves shortening and forcefully lengthening the body, as well as applying lateral forces to the walls to strengthen and widen the burrow. “A snake using concertina movements will make steady progress through a narrow channel or burrow by alternating waves of elongation and shortening,” the authors wrote, and the hagfish’s loose skin is well suited to such a strategy. The wriggling phase lasts until the burrowing hagfish sticks its head out of the substrate. It took the hagfishes an average of about seven minutes or more to complete their burrows.
Of course there are some caveats. The walls of the acrylic containers may have influenced the burrowing behavior in the laboratory or the final shape of the burrows. The authors recommend repeating the experiments using sediments from the natural habitat, implanting X-ray videography of hagfish with radio markers to record the movements. Body size and substrate type can also influence burrowing behavior. But overall, they believe their observations are “an accurate representation of how hagfish create and move around burrows in the wild.”
DOI: Journal of Experimental Biology, 2024. 10.1242/jeb.247544 (About DOIs).